Today's planet is Earth. Earth is the third planet from the sun, and at just the right distance to stay warm enough to have liquid water, but not so close to the sun to not have liquid water. In other words, it has liquid water, which seems to be very important for life, which is why Mars is looking so interesting recently. But I digress.
The human, boat, plane and house would be fine on most of the Earth, since they were built for Earth. But, let's say you wanted to make the Earth uninhabitable. Or, better yet, non-existent. Luckily for you, someone has made a list of plausible ways to destroy the Earth.
On to the Moon! The Moon is unusual among solar system moons in its relative size to it's primary. The most widely accepted explanation for the Moon's unusual size is that, approximately 4.5 billion years ago, a Mars-sized planet called Theia collided with Earth in a clanking blow, creating a field of debris from both Theia and Earth in orbit of Earth, which later coalesced into the Moon.
The color of the Moon is surprisingly deceptive, it looks like a light grey, but it's actually an asphalt color:
The gravity on the Moon is about one sixth of Earth's gravity. The plane wouldn't be able to fly, because of the lack of atmosphere. The boat wouldn't be very interesting, because the lunar "seas" or "mares" are made out of hardened basalt flows.
The human would need a spacesuit, because of the lack of oxygen, and the temperature, ranging from 242 degrees F (100 K) during the day to -280 degrees F (100 K) during the night. We know this is possible, because of the Apollo Moon landing program. The house shouldn't have too much trouble, but probably cycles of hot and cold and solar radiation would eventually wear it down.
The Moon is habitable enough that food and water could be a problem. Food would have to be brought with you, or farmed in a pressurized greenhouse. Water is a problem, because at the equator, water wouldn't last very long, not near the surface, anyway. However, at the lunar south pole, there are craters where sunlight never reaches the bottom. This is a likely location for water ice, because that strong evidence for water ice has been found there. Also, because sunlight never hits the bottom of the craters, a lunar base would have to deal with less temperature extremes.
That's it for the Earth and Moon, see you on Mars!
Tuesday, October 20, 2015
Monday, October 5, 2015
Project Apollo Archive
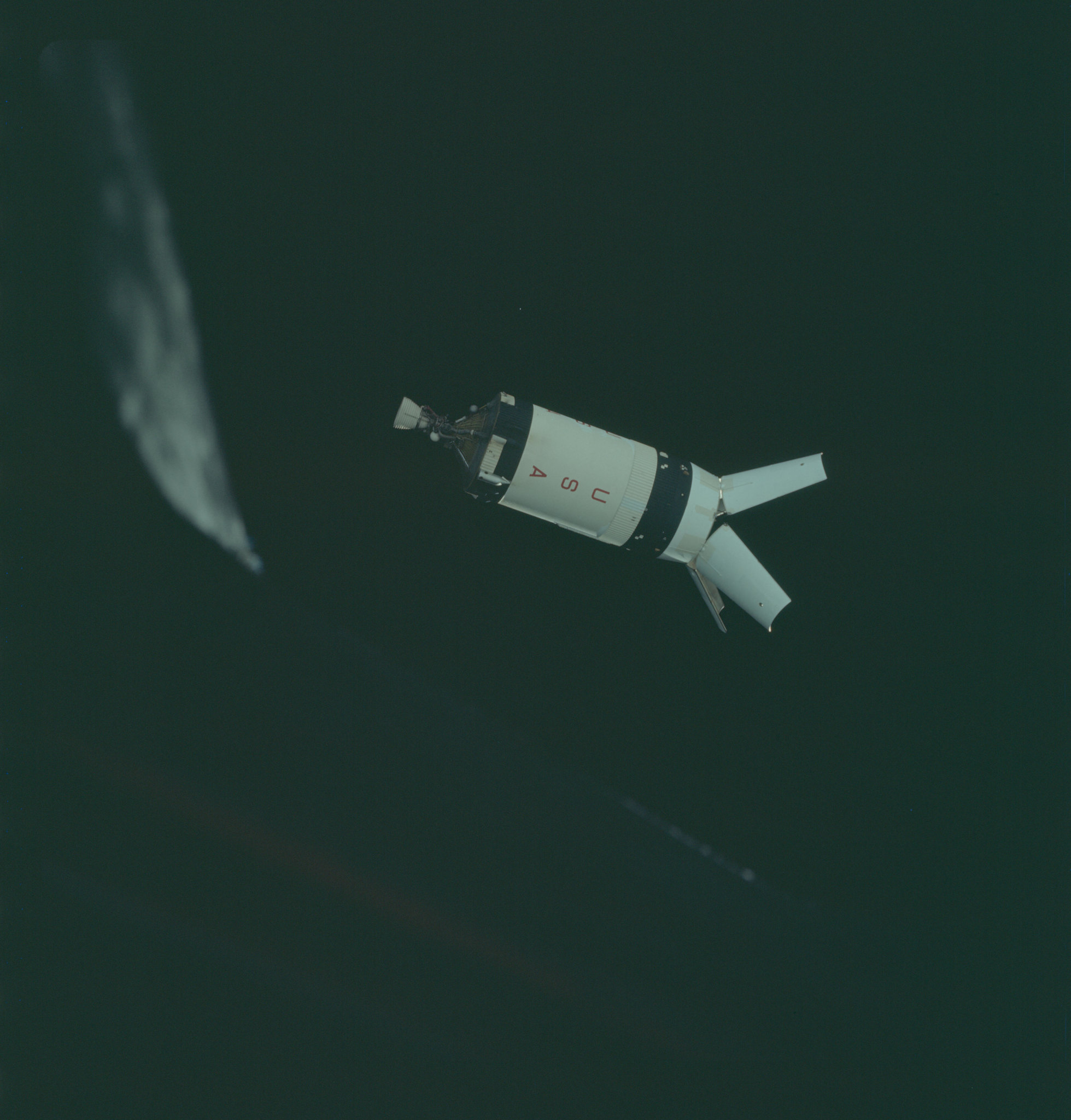
The Project Apollo Archive (Which has amazing images of spaceflight stuff) recently added a flickr gallery with over 10,000 HD scans from film taken during project Apollo. Enjoy.
Thursday, October 1, 2015
Rocket engines
Today we're going to look at rocket engines. Rocket engines are some of the most complex things in existence. They operate at incredible extremes, and yet they are very complex. Here's a test fire of a Merlin 1D rocket engine:
A rocket engine is very similar in concepts to a jet engine, with one difference: A jet engine collects oxidizer from the ambient air, but a rocket is completely self contained and self propelled. It carries all its fuel with it, so rocket engines have to combine fuel and oxidizer, ignite it, and propel it out at supersonic speeds.
The concepts behind rocket engines are simple, if not the implementations. A rocket is a lot like an inflated party balloon. The air in an inflated balloon is the fuel. It stretches the rubber skin, adding potential energy, and then is propelled by that energy out of the hole in the balloon, and the mass expelled from the balloon drives it across the room. Technically, a balloon is a pressure fed, monopropellant rocket.
There are a lot of kinds of rocket engines, but we'll start by looking at the most common kind: The liquid bipropellant rocket engine.
The fuel and oxidizer from the rocket's tanks are sucked from the tanks by high speed turbopumps. To give you an example, the J-2X hydrogen turbopump produces an incredible 16,000 horsepower. Where they go from there depends on the rocket. Somehow, the fuel is expanded to propel the turbo part of the turbopumps, either by being burnt with the oxidizer in a small combustion chamber and then sent to the turbopumps, if it's a gas generator cycle engine, sent around the engine to be heated up if it's an expander cycle engine, or fed through a pre-burner, which is very similar to a gas generator, except that the exhaust is fed back into the main combustion chamber to be burnt again after it's been used to power the turbopumps.
This is only a very basic overview of the different ways of dealing with turbopumps, for a more in-depth look at it see this blog's post. Actually, read all of the posts. No really, this is an great blog. All of the posts are interesting and relevant, especially to this post. Also, look at this video, from Copenhagen Suborbitals:
The fuel (and sometimes oxidizer) is then pumped through tubes in the walls of the main combustion chamber (MCC) and the nozzle walls. After that, it gets really interesting. The fuel and oxidizer is then combined and burnt in the MCC. The cold fuel flowing through the walls prevents the MCC from melting.
When fuel and oxidizer have been burnt in the MCC, the combustion products are forced out of the MCC, into the throat of the nozzle. This part is like that thing that you can put on the end of a garden hose to increase the fluid speed and decrease fluid pressure. This is known as the Venturi effect. However, the Venturi effect only works with subsonic fluids, if the fluid is supersonic, the flow "chokes" because the pressure waves that cause the Venturi effect no longer propagate upstream, instead, a diverging tube will increase speed and decrease pressure at supersonic speeds. This is the principle that makes the de Laval nozzle work, which is used in most liquid rocket engines. It is named after a Swedish inventor, Gustaf de Laval.
Here's a picture of a cross section of a de Laval nozzle:

"Laval-nozzle-(longitudinal-section-of-RD-107-jet-engine)" by Albina-belenkaya - Own work. Licensed under CC BY-SA 4.0 via Commons.
You can see how, after the exhaust has been accelerated to supersonic speeds by the nozzle converging from the MCC at the top of the picture, the nozzle diverges to further increase the speed.
Again, take a look at this blog for a more in-depth article about this.
Rocket engines are built differently for different environments. At sea level, engine nozzles are shaped differently than vacuum engine nozzles, because that the ambient pressure is higher, so nozzles need to be shaped differently to get the most thrust in the environment it's designed for.
Some rockets have hydraulic actuators which swivel the engine to provide steering while the engine's providing thrust, by changing the angle of thrust through the rocket. The hydraulic actuators are powered by the rocket engine itself. Here's a video of a J-2X engine being gimbal tested:
So how do you provide fuel to an engine that's rotating relative to the tanks? By using pipes with bellows. It seems pretty simple, except you have to remember, these pipes have less than -183 degrees Celsius liquid oxygen being forced through them, and, in some engines, even colder liquid hydrogen being pumped through them.
I'll finish this post with a video of a Copenhagen Suborbitals rocket being explained (This is a pressure fed, liquid chemical bi-propellent rocket engine):
Curious about what happened in the test? Look here.
A rocket engine is very similar in concepts to a jet engine, with one difference: A jet engine collects oxidizer from the ambient air, but a rocket is completely self contained and self propelled. It carries all its fuel with it, so rocket engines have to combine fuel and oxidizer, ignite it, and propel it out at supersonic speeds.
The concepts behind rocket engines are simple, if not the implementations. A rocket is a lot like an inflated party balloon. The air in an inflated balloon is the fuel. It stretches the rubber skin, adding potential energy, and then is propelled by that energy out of the hole in the balloon, and the mass expelled from the balloon drives it across the room. Technically, a balloon is a pressure fed, monopropellant rocket.
There are a lot of kinds of rocket engines, but we'll start by looking at the most common kind: The liquid bipropellant rocket engine.
The fuel and oxidizer from the rocket's tanks are sucked from the tanks by high speed turbopumps. To give you an example, the J-2X hydrogen turbopump produces an incredible 16,000 horsepower. Where they go from there depends on the rocket. Somehow, the fuel is expanded to propel the turbo part of the turbopumps, either by being burnt with the oxidizer in a small combustion chamber and then sent to the turbopumps, if it's a gas generator cycle engine, sent around the engine to be heated up if it's an expander cycle engine, or fed through a pre-burner, which is very similar to a gas generator, except that the exhaust is fed back into the main combustion chamber to be burnt again after it's been used to power the turbopumps.
This is only a very basic overview of the different ways of dealing with turbopumps, for a more in-depth look at it see this blog's post. Actually, read all of the posts. No really, this is an great blog. All of the posts are interesting and relevant, especially to this post. Also, look at this video, from Copenhagen Suborbitals:
The fuel (and sometimes oxidizer) is then pumped through tubes in the walls of the main combustion chamber (MCC) and the nozzle walls. After that, it gets really interesting. The fuel and oxidizer is then combined and burnt in the MCC. The cold fuel flowing through the walls prevents the MCC from melting.
When fuel and oxidizer have been burnt in the MCC, the combustion products are forced out of the MCC, into the throat of the nozzle. This part is like that thing that you can put on the end of a garden hose to increase the fluid speed and decrease fluid pressure. This is known as the Venturi effect. However, the Venturi effect only works with subsonic fluids, if the fluid is supersonic, the flow "chokes" because the pressure waves that cause the Venturi effect no longer propagate upstream, instead, a diverging tube will increase speed and decrease pressure at supersonic speeds. This is the principle that makes the de Laval nozzle work, which is used in most liquid rocket engines. It is named after a Swedish inventor, Gustaf de Laval.
Here's a picture of a cross section of a de Laval nozzle:

"Laval-nozzle-(longitudinal-section-of-RD-107-jet-engine)" by Albina-belenkaya - Own work. Licensed under CC BY-SA 4.0 via Commons.
You can see how, after the exhaust has been accelerated to supersonic speeds by the nozzle converging from the MCC at the top of the picture, the nozzle diverges to further increase the speed.
Again, take a look at this blog for a more in-depth article about this.
Rocket engines are built differently for different environments. At sea level, engine nozzles are shaped differently than vacuum engine nozzles, because that the ambient pressure is higher, so nozzles need to be shaped differently to get the most thrust in the environment it's designed for.
Some rockets have hydraulic actuators which swivel the engine to provide steering while the engine's providing thrust, by changing the angle of thrust through the rocket. The hydraulic actuators are powered by the rocket engine itself. Here's a video of a J-2X engine being gimbal tested:
So how do you provide fuel to an engine that's rotating relative to the tanks? By using pipes with bellows. It seems pretty simple, except you have to remember, these pipes have less than -183 degrees Celsius liquid oxygen being forced through them, and, in some engines, even colder liquid hydrogen being pumped through them.
I'll finish this post with a video of a Copenhagen Suborbitals rocket being explained (This is a pressure fed, liquid chemical bi-propellent rocket engine):
Curious about what happened in the test? Look here.
Labels:
ASL,
copsub,
efficiency,
engine,
fuel,
j-2x,
laval,
mcc,
merlin,
oxidizer,
propellent,
rocket
Subscribe to:
Posts (Atom)